Consider origin-centered circles $C(r)$ of radius $r \le 1$. I am seeking to learn how many rational points might lie on $C(r)$, where each rational point coordinate has height $\le h$. For example, these are the rationals in $[0,1]$ with $h \le 5$: $$ \left( 0,\frac{1}{5},\frac{1}{4},\frac{1}{3},\frac{2}{5},\frac{1}{2},\frac{3}{5}, \frac{2}{3},\frac{3}{4},\frac{4}{5},1 \right) $$ Rational points of height $\le h$ have both coordinates from this list, multiplied by $\pm 1$.
Q. What is the growth rate of the maximum number of rational points of height $\le h$ on $C(r)$, $r \le 1$, as a function of $h$?
Here is a bit of data up to $h=20$:
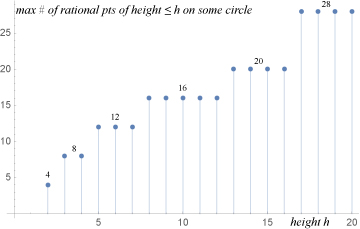
For example, for $h=7$, $C(\frac{5}{7})$ passes through these $12$ points: $$ \left( -\tfrac{4}{7} , -\tfrac{3}{7} \right), \left( -\tfrac{3}{7} , -\tfrac{4}{7} \right), \left( 0 , -\tfrac{5}{7} \right), \left( \tfrac{3}{7} , -\tfrac{4}{7} \right), \left( \tfrac{4}{7} , -\tfrac{3}{7} \right), \left( \tfrac{5}{7} , 0 \right), $$ $$ \left( \tfrac{4}{7} , \tfrac{3}{7} \right), \left( \tfrac{3}{7} , \tfrac{4}{7} \right), \left( 0 , \tfrac{5}{7} \right), \left( -\tfrac{3}{7} , \tfrac{4}{7} \right), \left( -\tfrac{4}{7} , \tfrac{3}{7} \right), \left( -\tfrac{5}{7} , 0 \right) $$ If I've calculated correctly, no circle passes through more than $12$ points of height $\le 7$. Circles that achieve these maxima are illustrated below.
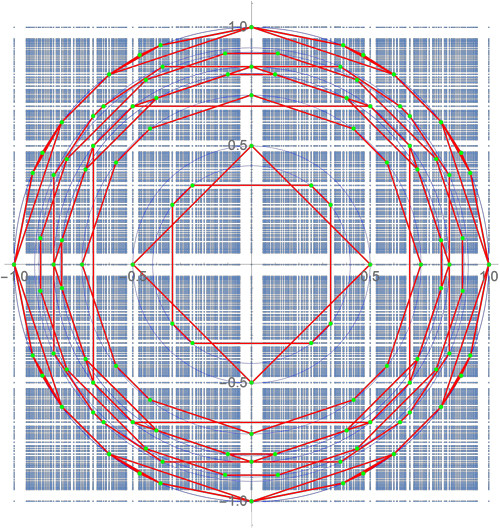
Background points are the rational points of height $h \le 20$.
Added. Since the radii that achieve the maxima I found for $13 \le h \le 20$ are all exactly $1$, it may be that the question can be reduced to counting the number of rational points of height $\le h$ on just specifically $C(1)$.
Answered. Lucia's answer matches even the small-$h$ data I gathered:
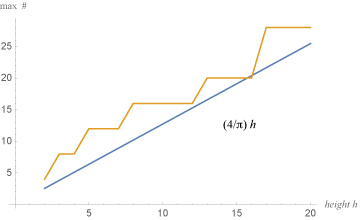