Solution (1).
Let $A=\{f\in A(\mathbb{D}):f(0)=f(1)\}$. It follows from the Maximum Modulus Principle that the Choquet boundary $Ch(A)$ does not contain $1$, as $B(A)$ and the set of peak points are same for this case. We claim that $Ch(A)=\mathbb{T}\setminus\{1\}$. It remains to prove that for any $z_0\in\mathbb{T}\setminus\{1\}$, $z_0$ is a peak point for $A$. Let us recall the Condition for the local Peak point.
A point $y\in K$ is said to be a local peak point of an Uniform algebra $A$ over $C(K)$ if for any open neighbourhood $U$ of $y$ and $\epsilon>0$, there exists $f \in A$ such that $\|f\| \leq 1$, $|f(y)|>1-\epsilon$ and
$|f|\leq \epsilon$ in $K \backslash U$.
It is known that if $K$ is metrizable then local peak point and peak point are the same.
Now choose $\epsilon>0$ and a neighborhood $U$ of $z_0$ in $\mathbb{T}$.
Case 1:When $|z_0-1|\geq 1$.
Get $f\in A(\mathbb{D})$ such that $|f(z_0)|>1-\frac{\epsilon}{2}$ and $|f|\lvert_{U^c}<\frac{\epsilon}{2}$.
Case 2:When $|z_0-1|<1$.
Get $f\in A(\mathbb{D})$ such that $|f(z_0)|>1-\frac{\epsilon}{2}|z_0-1|$ and $|f||_{U^c}<\frac{\epsilon}{2}|z_0-1|$.
Now define $g(z)=\frac{z(z-1)}{|z_0-1|}.f(z)$. Clearly $g\in A$ and $|g(z_0)|>1-\epsilon$, $|g||_{U^c}<\epsilon$.
This shows $z_0$ is a peak point for $A$ and hence $Ch(A)=\mathbb{T}\setminus\{1\}$.
Solution (2)
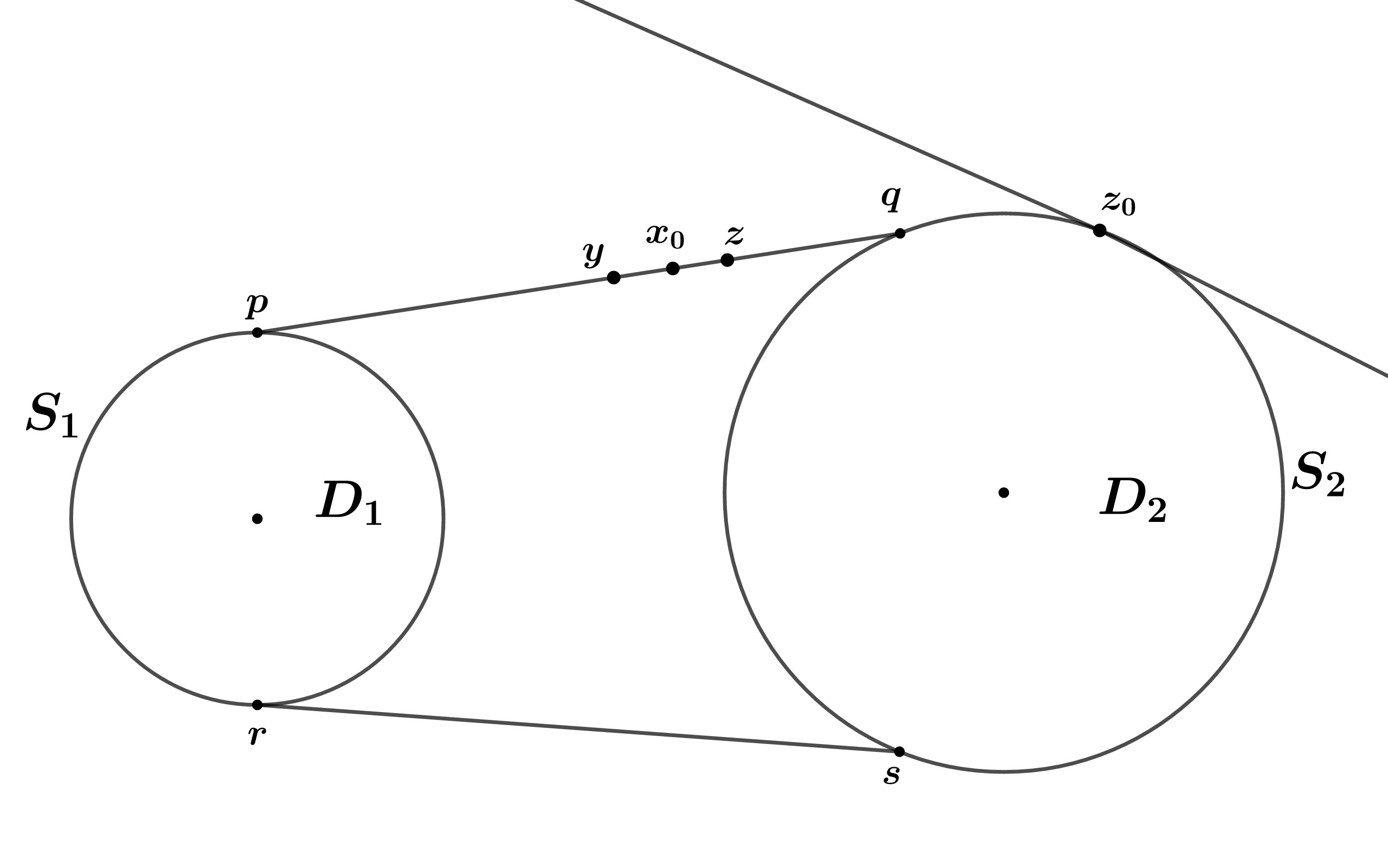
Let $K$ be the subset of the plane consisting of the convex hull of two disjoint circles and let $M$ be the complex-valued
affine functions on $K$. Let the four tangent points be $p,q,r,s$, denote the open arcs $pr$ and $qs$ as $S_{1}$ and $S_{2}$ and denote the smaller circle as
$D_{1}$ and the bigger circle as $D_{2}$.
Let $x_{0}$ be any point on the open line segment joining $p$ and $q$. Then there exists distinct points $y$ and $z$ on the line segment such that $x_{0} = \frac{y+z}{2}$. For any $f \in M$,
$f(x_{0}) =\frac{f(y)+f(z)}{2}$; this implies $f(x_{0}) = \int_{K} fd(\frac{\delta_{y}+\delta_{z}}{2})$, but $\delta_{x_{0}} \neq \frac{\delta_{y}+\delta_{z}}{2}$. Hence, $x_{0}\notin Ch(M)$ and hence is not a peak point for $M$.
Consider any $z_{0} \in S_{1}\cup S_{2}$ and the tangent line say $ax+by=c$ for some $a,b,c \in \mathbb{R}$ to the point $z_{0}$ as shown in the above
figure. Then $K \subset \{x+iy \in \mathbb{C}: ax+by \leq c\}$ and $g : \mathbb{C} \rightarrow \mathbb{R}$ defined as $g(x+iy)=ax+by$ is a real linear functional. Without loss of generality assume that $c>0$. Hence,
$\tilde{g}(z)=g(z)-ig(iz)$, is a complex linear functional. Now $\sup_{z\in K}|\tilde{g}(z)|=\sup_{z\in K}|g(z)|$. Hence the maximum of $\tilde{g}$ is same as that of $g$. Now $\tilde{g}(z_0)=|\tilde{g}(z_0)|e^{i\theta}$, ie. $e^{-i\theta}\tilde{g}(z_0)=|\tilde{g}(z_0)|\geq|g(z_0)|=\sup_K g(z)=c$. Consider the linear functional $e^{-i\theta}\tilde{g}(z)$ which is affine on $K$, $|e^{-i\theta}\tilde{g}(z)|=|\tilde{g}(z)|\leq c$.
Hence, $z_{0}$ is a peak point for $M$.
Now suppose $p$ is not in the Choquet boundary for $M$. This implies
$\delta_{p} \not\in ext(K(M))$. Therefore there exists $z_{1},z_{2} \in S_{1} \cup S_{2}$ such that $\delta_{p} = \frac{\delta_{z_{1}}+\delta_{z_{2}}}{2}$. Thiis implies
$p=\frac{z_{1}+z_{2}}{2}$ which is a contradiction since $p$ lies on the boundary of the circle. This implies $p$ is in $Ch(M)$. Similarly, the remaining tangent
points also lie in the Choquet boundary for $M$.
Suppose $p$ is a peak point for $M$. Then we will get $f \in M$ such that $|f(x)|<|f(p)|$, for $x \in K\setminus\{p\}$. Now $f(p)=|f(p)|e^{i\theta}$, define $h(z)=Re(e^{-i\theta}f(z))$, then $|h(z)|\leq |f(z)|$, for all $z\in\mathbb{C}$ and $h(p)=|f(p)|=sup_K|f(z)|$. That is $h$ is a real linear functional on $\mathbb{C}$ which attains its supremum over a smooth surface $K$ at $p$. It shows that $h$ must be the tangent to the surface. Hence $h$ must coincides with the line segment $pq$. That is $|f(p)|=h(p)=h(q)\leq|f(q)|<|f(p)|$, a contradiction. Hence such an $f$ does not exist.
This implies $D_{1} \subset \{x \in \mathbb{R}^{2}: f(x)\leq \|f\|\}$ and
$f(x) = \|f\|$ is the tangent line to $D_{1}$ at point $p$. This implies $f(x) = \|f\|$, for all $x$ on the line segment joining $p$ and $q$, which is a contradiction.
Hence, $p$ is not a peak point for $M$.
Therefore, we get that $Ch(M)$ is precisely $S_{1} \cup S_{2}\cup \{p,q,r,s\}$ and $Ch(M)\setminus \{p,q,r,s\}$ is the set of peak points for $M$.