We know that $0 \subseteq V_n \subseteq \mathbb{C}^n \cong \mathbb{1}_n \oplus V_n$ is a composition series for the natural $\mathbb{C}[S_n]$-module $\mathbb{C}^n$ for all $n \geq 2$. Now we have compatible inclusions $S_n \subseteq S_{n+1}$, $\mathbb{C}^n \subseteq \mathbb{C}^{n+1}$, $V_n \subseteq V_{n+1}$, which gives $$ 0 \subseteq \bigcup_n V_n \subseteq \bigcup_n\mathbb{C}^n =: \mathbb{C}^\infty $$ as $S_\infty := \bigcup_n S_n$-modules. In fact, it can be easily argued that $\bigcup_n V_n$ — the vectors whose coefficients sum to $0$ — is the only proper submodule of $\mathbb{C}^\infty$. Hence the length of $\mathbb{C}^\infty$ agrees with the length of $\mathbb{C}^n$ for $n$ big enough.
More generally, for $k$ fixed, the length of $(\mathbb{C}^n)^{\otimes k} \cong (\mathbb{1}_n \oplus V_n)^{\otimes k} \cong \bigoplus_{0 \leq i \leq k} \binom{k}{i} V_n^{\otimes i}$ stabilises for $n \geq 2k$ (and the multiplicities are given in terms of the stable Kronecker coefficients). On the other hand, Sam and Snowden's paper Stability patterns in representation theory as referred to by this answer to Specht modules for symmetric group $S_{\infty}$ proves that $(\mathbb{C}^\infty)^{\otimes k}$ has finite length as well. In fact, I believe an elementary argument shows that here the length of the infinite module is bounded above by the length of the finite one: if $0 \subsetneq M_1 \subsetneq \dots \subsetneq M_l = (\mathbb{C}^n)^{\otimes k}$, we can pick $l$ vectors $v_i \in M_i \setminus M_{i-1}$ witnessing the strictness of inclusions; then by picking $N$ big enough to accommodate all the $l$ vectors, we have $$0 \subsetneq \mathbb{C}[S_N] \cdot v_1 \subsetneq \mathbb{C}[S_N] \cdot v_1 + \mathbb{C}[S_N] \cdot v_2 \subsetneq \dotsb \subsetneq \sum_{1 \leq i \leq l} \mathbb{C}[S_n] \cdot v_i \subseteq (\mathbb{C}^N)^{\otimes k}$$ which shows that $l \leq \operatorname{length} (\mathbb{C}^N)^{\otimes k}$. My question is: do we have equality?
For $k = 2$, I worked out two composition series
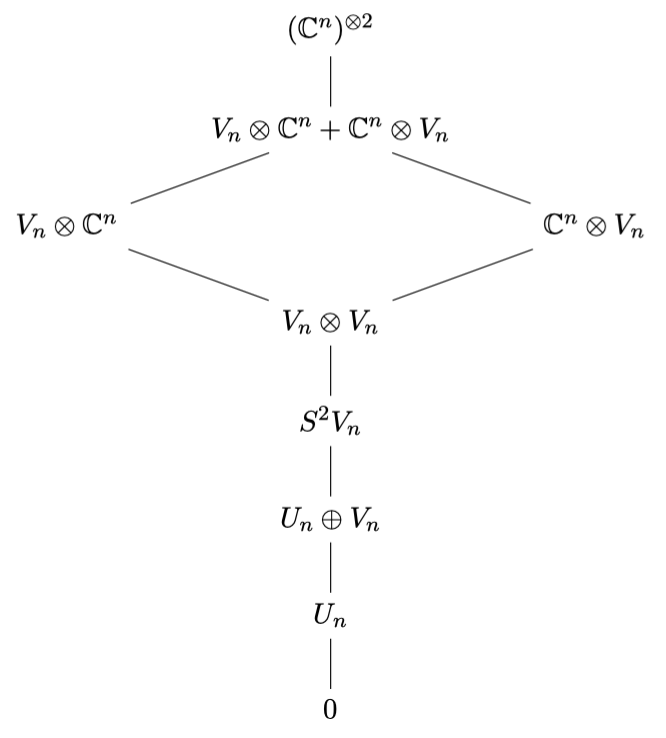
which have inductive limits in $(\mathbb{C}^\infty)^{\otimes k}$, as I found generators in $\mathbb{C}^4 \otimes \mathbb{C}^4$ for each subspace: for example, if we write $\mathbb{C}^n = \bigoplus_i \mathbb{C} e_i$,
- $U_n = \mathbb{C}[S_n] \cdot (e_1 e_2 + e_2 e_3 + e_3 e_4 + e_4 e_1)$ where $e_i e_j := \frac{1}{2} (e_i \otimes e_j + e_j \otimes e_i)$ for all $n \geq 4$;
- $S^2 V_n = \mathbb{C}[S_n] \cdot (e_1 e_1 - e_1 e_2 + e_2 e_2)$ — viewing $e_i \otimes e_j$ as an edge $i \to j$, this space consists precisely of the weighted undirected graphs in $n$ vertices (possibly with loops) where at each vertex the edge weights sum to $0$;
- $V_n \otimes \mathbb{C}^n = \mathbb C [S_n] \cdot (e_1 \otimes e_1 - e_1 \otimes e_2)$ consists precisely of weighted directed graphs where at each vertex, the outgoing edge weights sum to $0$.
Therefore, the infinite length and the finite length agree for $k = 2$ as well.
Of course this method of ‘finding a chain and hoping that it lifts to a chain for $S_\infty$’ is very elementary and ad hoc. Are there any machineries I can use?