Partial answer
Concerning the particular example in the question, $-c_{\{1\}}$ is conjugate to $c_{\{1,3,4,5\}}$, and $-c_{\{5\}}$ is conjugate to $-c_{\{1,2,3,4\}}$. This can almost be deduced from the dimension of the -1-eigenspaces, but with the following twist. The Dynkin diagram of type $A_{10}$ “folds” onto the Dynkin diagram of type $C_5$. This “folding” induces a surjection of corresponding sets of simple roots
$
\pi\colon \Sigma_{A_{10}} \twoheadrightarrow \Sigma_{B_5}
$
and an associated embedding
$$
\iota\colon W(C_{5}) \hookrightarrow W(A_{10})
$$
of the Weyl group of type $C_5$ into the Weyl group of type $A_{10}$. (See this question.) The embedding sends $-1\in W(C_{5})$ to the longest element $w_0\in W(A_{10})$, and its image is the subgroup $ W_0 \subset W(A_{10})$ of elements that commute with $w_0$. More generally:
Claim 1: The embedding $\iota$ sends the longest word $w_I = c_I$ of the parabolic subgroup associated with a subset of simple roots $I\subset \Sigma_{B_5}$ to the longest word $w_{\pi^{-1}I}$ of the parabolic subgroup corresponding to the "unfolded" subset $\pi^{-1}I\subset \Sigma_{A_{10}}$.
We thus obtain a very explicit bijection between $\mathcal C := $ {conjugacy classes of involutions in $W(C_{5})$} and $\mathcal A := $ {$W_0$-conjugacy classes of involutions in $W_0$}:
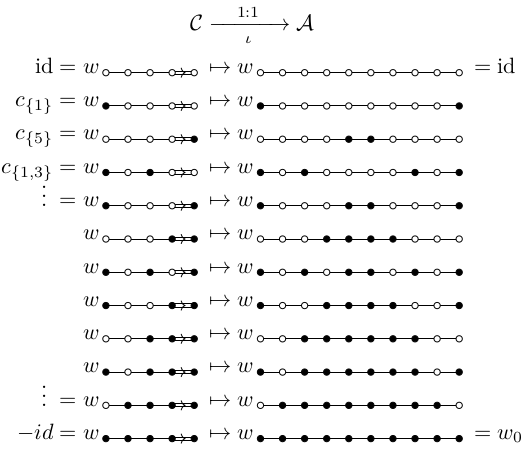
Under this bijection, multiplication with $-1$ on $\mathcal C$ corresponds to multiplication with $w_0$ on $\mathcal A$.
Now let $l^-(w)$ denote the dimension of the -1-eigenspace of $w$. On the representatives of involutions $w$ listed above, the tuple $((l^-(w),l^-(\iota w))$ takes the following values:
$(0,0)$,
$(1,2)$,
$(1,1)$,
$(2,4)$,
$(2,3)$,
$(2,2)$,
$(3,5)$,
$(3,4)$,
$(3,3)$,
$(4,4)$,
$(4,3)$,
$(5,5)$.
These are all distinct! Therefore, $-w$ (for $w\in \mathcal C$) and $w_0w$ (for $w\in\mathcal A$) are uniquely determined by the tuple $(l^-(-w),l^-(w_0w))$.
Claim 2: By computing the tuples $(l^-(-w),l^-(w_0w))$, we find that:
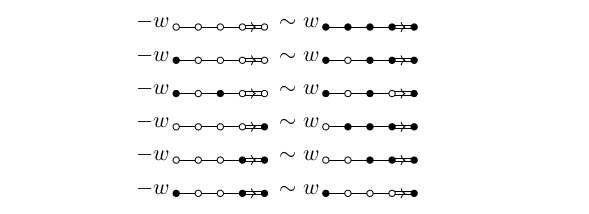
The second and fourth line answer the question.
Generalizations
It is straightforward to extend this analysis to $W(B_n) = W(C_n)$ for arbitrary $n$, and to $W(F_4)$ (using the folding of $E_6$ onto $F_4$). With some more care, the conjugacy class of $-c_I$ can also be computed for almost all $I$ for $W(D_{2n})$, but with a remaining ambiguity concerning the involutions corresponding to:

I do not know how to treat $W(E_7)$ and $W(E_8)$.
Details
Claim 1 follows from the explicit description of foldings in the first section of Steinberg, Endomorphisms of Linear Algebraic Groups (1968); see in particular 1.12(c), 1.32(a) and Corollary 1.33. I would be interested whether there is a more direct, group theoretic argument for this claim.
For the calculation of $l^-(w_0w_I)$ that is implicit in claim 2, decompose the ambient vector space into the span of $I$ and its orthogonal complement, and observe that the restriction of $w_I$ to the complement is trivial.